BLOG - SPECIAL STEAM TURBINES
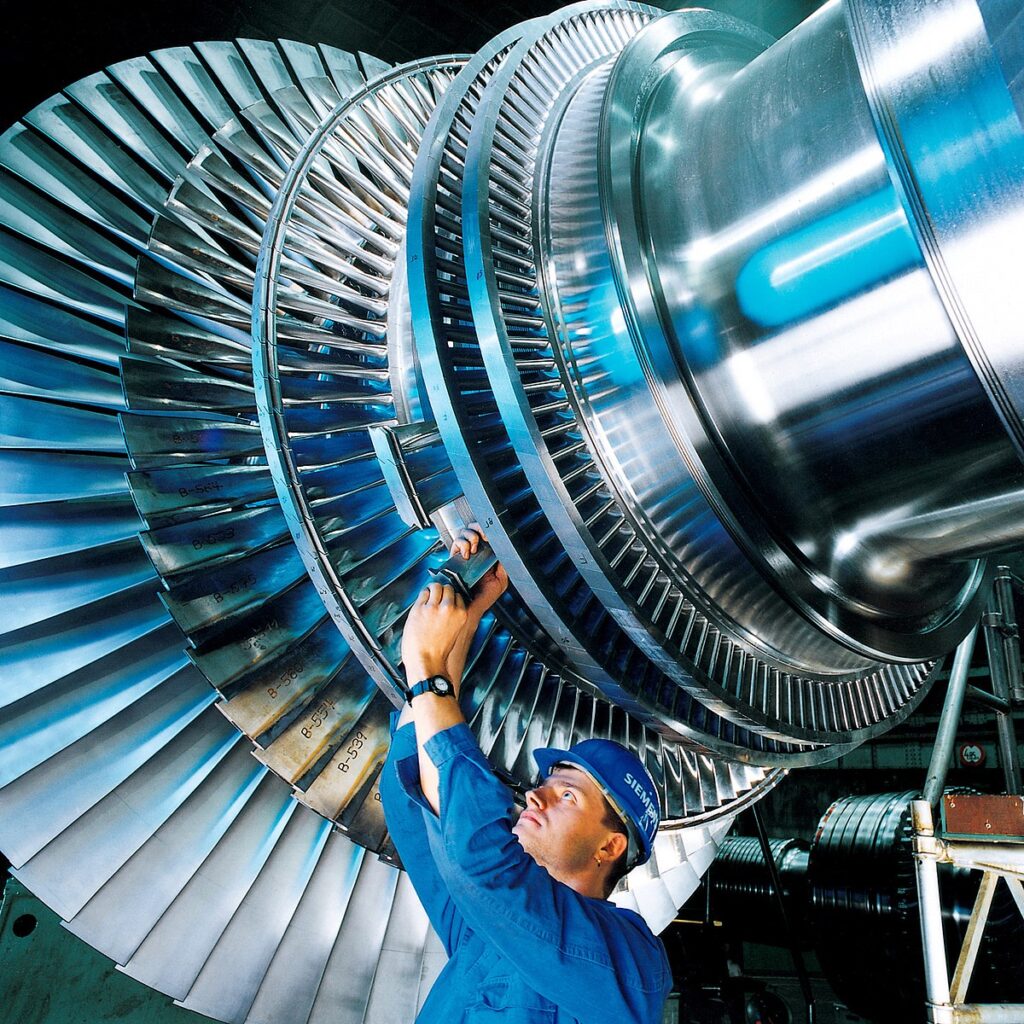
DESIGN & ENGINEERING
HIGH PERFORMANCE
HIGH RELIABILITY
To achieve high quality in design and engineering of industrial steam turbines in oil, gas, and power generation plants, the following actions can be taken:
Detailed specifications: A comprehensive set of specifications should be developed to clearly define the requirements and expectations for the steam turbine. This includes the power output, steam pressure and temperature, operating conditions, and any special features or requirements.
Advanced design tools and techniques: Advanced design tools and techniques, such as computational fluid dynamics (CFD) and finite element analysis (FEA), should be used to optimize the design and ensure that it meets the required specifications and standards.
Experienced and qualified engineers: The design and engineering of the steam turbine should be led by experienced and qualified engineers who have a deep understanding of the technology, the operating environment, and the relevant standards and regulations.
Compliance with industry standards and regulations: The design and engineering of the steam turbine should comply with industry standards and regulations, including codes such as ASME, API, and ISO, as well as regulatory requirements from government agencies such as the EPA, OSHA, and NRC.
Robust testing and validation: The design and engineering of the steam turbine should be subject to rigorous testing and validation to ensure that it meets the required specifications and standards. This includes testing of individual components, as well as the assembled steam turbine.
Continuous improvement: Continuous improvement processes should be implemented to identify and address areas for improvement in the design and engineering of the steam turbine. This includes gathering feedback from operators and maintenance personnel, monitoring performance metrics, and incorporating lessons learned from past incidents.
Collaboration and communication: Collaboration and communication between the design and engineering team, as well as with other stakeholders such as operators and maintenance personnel, is essential to ensure that the steam turbine is designed and engineered to meet the needs of all parties and to ensure that it is safe, reliable, and efficient.
By taking these actions, the quality of the design and engineering of industrial steam turbines in oil, gas, and power generation plants can be maximized, ensuring that they meet the required specifications, operate safely and efficiently, and minimize downtime and maintenance costs.
To achieve high performance in industrial steam turbines in oil, gas, and power generation plants, the following actions can be taken:
Optimize design: The steam turbine should be designed and optimized for the specific application, taking into account the operating conditions, environmental factors, and potential challenges. This includes selecting appropriate materials, components, and manufacturing processes, and incorporating redundancy and fail-safe features.
Efficient operation: The steam turbine should be operated efficiently, taking into account the steam flow rate, temperature, and pressure. This includes proper maintenance of steam traps, condensate systems, and other supporting equipment to ensure that the steam is delivered to the turbine in optimal condition.
Regular maintenance: A comprehensive maintenance program should be established and implemented to ensure the steam turbine operates efficiently and reliably over its lifetime. This includes regular inspections, monitoring, and testing, as well as timely repairs and replacement of components when necessary.
Monitoring and diagnostics: Advanced monitoring and diagnostic systems can be used to detect potential issues and provide early warning of impending failures. This allows for proactive maintenance and repairs, minimizing downtime and reducing the risk of catastrophic failure.
Upgrades and retrofits: Upgrades and retrofits can be implemented to improve the performance of existing steam turbines. This includes the installation of new components, such as blades, seals, or bearings, as well as the implementation of new control systems or software to optimize the operation of the turbine.
Collaboration and communication: Collaboration and communication between the design and engineering team, as well as with other stakeholders such as operators and maintenance personnel, is essential to ensure that the steam turbine is operated and maintained to maximize its performance.
Continuous improvement: Continuous improvement processes should be implemented to identify and address areas for improvement in the design, operation, and maintenance of the steam turbine. This includes gathering feedback from operators and maintenance personnel, monitoring performance metrics, and incorporating lessons learned from past incidents.
By taking these actions, industrial steam turbines in oil, gas, and power generation plants can achieve high performance, maximizing power output, efficiency, and reliability, while minimizing downtime and maintenance costs.
To achieve high reliability in industrial steam turbines in oil, gas, and power generation plants, the following actions can be taken:
Design for reliability: The steam turbine should be designed with reliability in mind, taking into account the operating conditions, environmental factors, and potential failure modes. This includes selecting appropriate materials, components, and manufacturing processes, and incorporating redundancy and fail-safe features.
Quality control: The manufacturing process should be subject to rigorous quality control measures, including inspection and testing of materials, components, and finished products. This ensures that the steam turbine meets the required specifications and standards.
Regular maintenance: A comprehensive maintenance program should be established and implemented to ensure the steam turbine operates efficiently and reliably over its lifetime. This includes regular inspections, monitoring, and testing, as well as timely repairs and replacement of components when necessary.
Monitoring and diagnostics: Advanced monitoring and diagnostic systems can be used to detect potential issues and provide early warning of impending failures. This allows for proactive maintenance and repairs, minimizing downtime and reducing the risk of catastrophic failure.
Training and expertise: Operators and maintenance personnel should receive adequate training and have the necessary expertise to operate and maintain the steam turbine. This includes knowledge of the equipment, operating procedures, and safety requirements.
Continuous improvement: Continuous improvement processes should be implemented to identify and address areas for improvement in the design, manufacturing, operation, and maintenance of the steam turbine. This includes gathering feedback from operators and maintenance personnel, monitoring performance metrics, and incorporating lessons learned from past incidents.
Compliance with industry standards and regulations: Compliance with industry standards and regulations ensures that the steam turbine meets the required safety and performance standards. This includes adherence to industry codes and standards such as ASME, API, and ISO, as well as compliance with regulatory requirements from government agencies such as the EPA, OSHA, and NRC.
By taking these actions, industrial steam turbines in oil, gas, and power generation plants can achieve high reliability, minimizing downtime, reducing maintenance costs, and ensuring safe and efficient operation
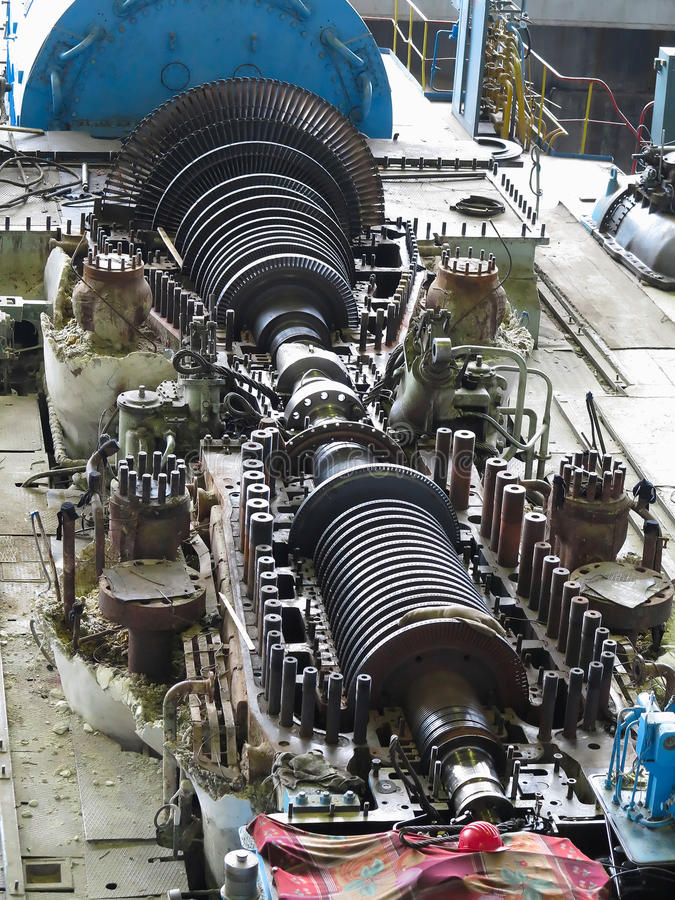
GOVERNOR CONTROL SYSTEM
TURBINE BLADES
ROTOR & SHAFT
Response Time: The governor control system should respond quickly and accurately to changes in the load demand to maintain the desired speed and power output of the turbine. The response time is critical for preventing over-speed or under-speed conditions that can cause damage to the turbine and associated equipment.
Stability: The governor control system must be stable under all operating conditions to prevent oscillations or hunting that can cause instability and damage to the turbine. Stability is particularly critical during load changes, which can cause transient effects in the turbine system.
Accuracy: The governor control system must be accurate in maintaining the desired speed and power output of the turbine to ensure efficient operation and prevent damage to the turbine. Accurate speed control is particularly important in steam turbines that operate at high speeds and generate high power output.
Redundancy: The governor control system should have redundancy in critical components such as sensors, actuators, and controllers to ensure continued operation in the event of a component failure. Redundancy is particularly important in steam turbines that are used for critical applications such as power generation in nuclear plants.
Calibration: The governor control system must be calibrated periodically to ensure accurate and reliable operation. Calibration should be performed by trained personnel using calibrated equipment to ensure accurate measurements and prevent errors in the control system.
Maintenance: The governor control system must be maintained regularly to ensure reliable operation and prevent component failures. Maintenance activities should include inspections, testing, and replacement of worn or damaged components.
Control Algorithm: The control algorithm used in the governor control system should be designed to provide stable and accurate control under all operating conditions. The algorithm should be optimized for the specific turbine and load characteristics to ensure efficient and reliable operation
Aerodynamics: The design of turbine blades should be optimized to maximize the aerodynamic efficiency of the turbine. This involves shaping the blade profile and controlling the flow of steam over the blade surfaces to minimize losses due to turbulence and separation.
Mechanical Integrity: The design of turbine blades should be structurally sound to withstand the high temperatures, pressures, and stresses that occur during operation. Blade materials, cooling systems, and attachment methods should be selected and designed to ensure reliable and safe operation.
Manufacturing Tolerances: The design of turbine blades must take into account manufacturing tolerances to ensure consistent performance and prevent blade failure due to dimensional errors. Close tolerances and precision manufacturing techniques may be required to achieve the desired blade geometry and performance.
Material Selection: The selection of materials for turbine blades is critical to ensuring long-term reliability and performance. Blade materials must be able to withstand the high temperatures and stresses that occur during operation while maintaining their mechanical properties and resistance to corrosion.
Thermal Stress: The design of turbine blades should minimize thermal stresses that can occur due to temperature gradients across the blade. This can be achieved through the use of cooling systems, thermal barrier coatings, and careful design of the blade profile and materials.
Blade Vibration: The design of turbine blades must take into account the potential for blade vibration due to aerodynamic and mechanical forces. The blade profile, damping systems, and attachment methods must be optimized to minimize vibration and prevent damage to the turbine.
Maintenance and Replacement: The design of turbine blades should take into account the need for periodic maintenance and replacement over the life of the turbine. Blade attachment methods and access for inspection and replacement should be designed to minimize downtime and cost
Mechanical Integrity: The design of the rotor and shaft must ensure that they can withstand the high temperatures, pressures, and stresses that occur during operation. Materials, dimensions, and manufacturing methods must be carefully selected and optimized to ensure that the rotor and shaft are structurally sound and can operate safely and reliably.
Fatigue Resistance: The design of the rotor and shaft must take into account the potential for fatigue failure due to cyclic loading during operation. This requires careful consideration of material selection, manufacturing processes, and design features such as fillets, radii, and surface finishes.
Dynamic Balancing: The design of the rotor and shaft must take into account the potential for unbalanced forces and vibration during operation. Dynamic balancing techniques may be required to ensure smooth and stable operation of the turbine.
Alignment: The design of the rotor and shaft must ensure that they are aligned correctly with other components of the turbine, such as the bearings and seals. Misalignment can cause excessive wear and damage to these components, leading to reduced efficiency and reliability.
Thermal Stress: The design of the rotor and shaft must take into account the potential for thermal stresses due to temperature gradients across the rotor and shaft. Thermal expansion and contraction can cause distortion and fatigue failure if not managed carefully through material selection and design features such as keyways and couplings.
Manufacturing Tolerances: The design of the rotor and shaft must take into account manufacturing tolerances to ensure consistent performance and prevent failure due to dimensional errors. Close tolerances and precision manufacturing techniques may be required to achieve the desired geometry and performance.
Maintenance and Replacement: The design of the rotor and shaft must take into account the need for periodic maintenance and replacement over the life of the turbine. Access for inspection and replacement should be designed to minimize downtime and cost. The design should also take into account the potential for upgrades and modifications to the turbine over its lifetime
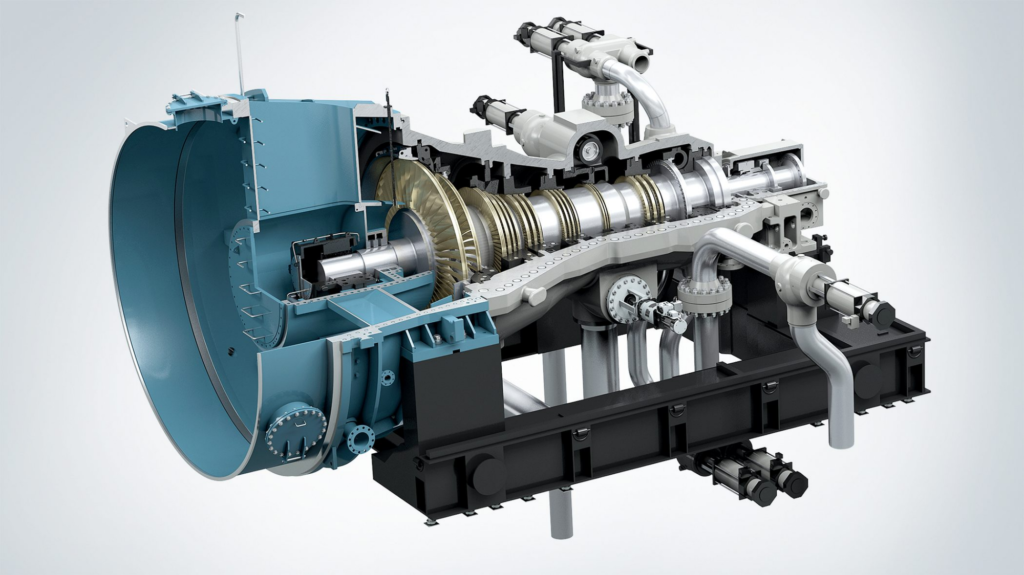
CONTROL & MONITORING SYSTEM
EMERGENCY SHUTDOWN SYSTEM
WATER & VACUUM SEAL SYSTEM
Control System Architecture: The control system architecture must be carefully designed to ensure optimal performance, reliability, and flexibility. The architecture should allow for seamless integration with other turbine components and systems, such as the generator, governor, and monitoring systems.
Control Algorithms: The control algorithms used in the control system must be carefully designed and optimized to ensure that the turbine operates safely and efficiently under all operating conditions. The algorithms should be able to respond quickly to changes in load demand and other operating parameters.
Monitoring and Diagnostics: The control system should include comprehensive monitoring and diagnostic capabilities to detect potential problems early and prevent catastrophic failures. The monitoring system should be able to collect and analyze data on a range of operating parameters, including vibration, temperature, and pressure, and provide timely alerts and notifications if anomalies are detected.
HMI and Visualization: The control system should provide an intuitive and user-friendly HMI (human-machine interface) to allow operators to monitor and control the turbine easily. The visualization system should provide clear and concise displays of critical turbine operating parameters and enable operators to quickly identify potential issues.
Cybersecurity: The control system should be designed to be secure and resilient against cyber attacks. This requires the implementation of robust cybersecurity measures, such as firewalls, intrusion detection systems, and access controls.
Redundancy and Backup: The control system should include redundancy and backup capabilities to ensure that the turbine can continue to operate safely and reliably in the event of a system failure. This may include backup control systems, redundant sensors and actuators, and backup power supplies.
Maintenance and Upgrades: The control system should be designed to be easily maintainable and upgradeable over the life of the turbine. This requires the use of standardized components, open interfaces, and modular design principles
Rapid Response: The emergency shutdown system must be designed to respond quickly and reliably to emergency situations, such as turbine overspeed, loss of oil pressure, or other critical failures.
Redundancy: The emergency shutdown system should include redundant components and backup systems to ensure that it can operate reliably under all operating conditions. This may include redundant sensors, actuators, and control circuits.
Safety: The emergency shutdown system should be designed to ensure the safety of personnel and equipment. This may include features such as automatic fire suppression systems or emergency ventilation systems.
Testing and Maintenance: The emergency shutdown system must be regularly tested and maintained to ensure that it operates as designed when an emergency occurs. This may involve routine inspections, functional testing, and periodic upgrades and replacements.
Integration with Other Systems: The emergency shutdown system must be integrated with other turbine control systems, such as the governor and monitoring systems, to ensure that it operates seamlessly with the rest of the turbine.
Human-Machine Interface: The emergency shutdown system should include an intuitive and easy-to-use human-machine interface (HMI) to allow operators to quickly initiate a shutdown in an emergency situation.
Documentation and Training: The emergency shutdown system should be well-documented, and operators and maintenance personnel should be trained on its operation and maintenance procedures
Proper Design: The water or vacuum seal system must be properly designed to prevent steam from leaking into the condenser and to prevent air from entering the turbine casing. This requires careful selection of the seal type, dimensions, and material.
Corrosion Resistance: The seal system must be designed to resist corrosion from the cooling water and condensate. This may require the use of corrosion-resistant materials, such as stainless steel or titanium, and the use of coatings or other protective measures.
Monitoring: The water or vacuum seal system should be equipped with appropriate monitoring equipment, such as pressure sensors and temperature gauges, to detect any leaks or other issues. The data from these instruments should be regularly analyzed to identify any issues and allow for proactive maintenance and repairs.
Maintenance: The water or vacuum seal system requires regular maintenance and inspection to detect any signs of wear, corrosion, or other damage that may affect its performance. This may include checking for leaks, replacing worn components, and lubricating the seals.
Alignment: The water or vacuum seal system must be properly aligned with the turbine shaft to ensure that it operates smoothly and without any unnecessary vibration or wear. Misalignment can cause seal failure and other issues, leading to reduced turbine efficiency and reliability.
Water Quality: The quality of the cooling water used in the seal system is critical. Poor water quality can cause scaling, corrosion, and other issues that can affect the performance of the seals and other turbine components. Proper water treatment and filtration are essential to maintain water quality.
Proper Start-up and Shutdown Procedures: The water or vacuum seal system requires proper start-up and shutdown procedures to ensure that it operates correctly and without any unnecessary wear or damage. The procedures should include steps to properly warm up the seals, align them with the turbine shaft, and ensure that they are properly lubricated and cooled.
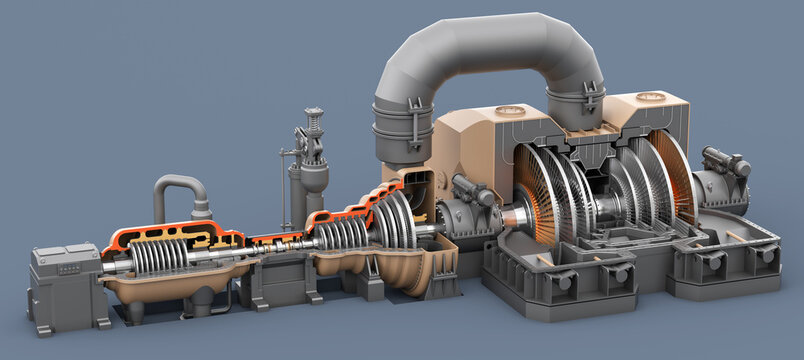