HOW TO IMPROVE THE STEAM RATE, POWER DENSITY & THERMAL EFFICIENCY IN SPECIAL STEAM TURBINES
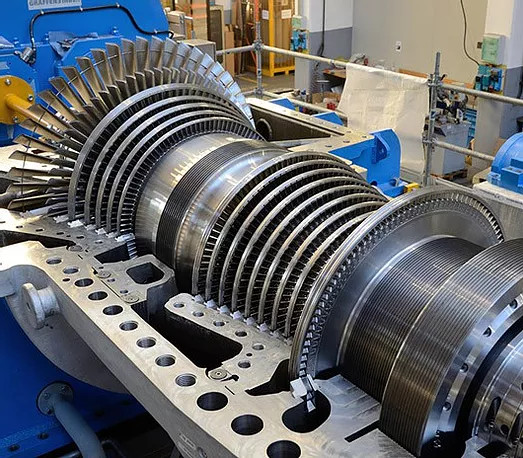
LIMITATIONS IN ENGINEERING & DESIGN TO IMPROVE THE STEAM RATE, POWER DENSITY & THERMAL EFFICIENCY IN STEAM TURBINES
Material Limitations:
- The selection of materials with improved properties, such as higher temperature and pressure capabilities, can be limited by cost, availability, and manufacturing constraints.
- Higher temperatures and pressures can accelerate material degradation mechanisms, including creep, fatigue, and corrosion, which may limit the operating range and service life of components.
- Introducing new materials or coatings may require extensive research, development, and testing to ensure their compatibility and reliability in steam turbine applications.
Design Constraints:
- Existing turbine designs may have limitations that prevent significant improvements in steam rate, thermal efficiency, and power density without major modifications or complete redesign.
- Retrofitting or upgrading existing turbines to incorporate advanced technologies may be challenging due to space constraints, compatibility issues, or the need for extensive modifications to the existing infrastructure.
Turbine Dynamics and Stability:
- Higher steam rates and thermal efficiencies can lead to changes in the dynamic behavior and stability of the turbine, including increased vibration levels and potential for resonance.
- The introduction of advanced blade designs or higher steam velocities may require additional considerations for blade flutter, blade-tip clearance, and aerodynamic stability.
Operating Conditions:
- Achieving higher steam rates and thermal efficiencies often requires operating the turbine under more demanding conditions, such as higher temperatures and pressures.
- Operating at higher conditions may increase the risk of component failures, reduce the margin for safety, and necessitate more frequent inspections, maintenance, and monitoring.
Environmental Considerations:
- Improving thermal efficiency and power density may require higher combustion temperatures, which can result in increased emissions of pollutants, including nitrogen oxides (NOx).
- Stricter environmental regulations may impose limitations on the allowable emissions, requiring the use of additional emission control technologies or compromises in performance optimization.
Cost and Economic Viability:
- Implementing advanced technologies or design enhancements to improve steam rate, thermal efficiency, and power density can be costly, requiring significant investment in research, development, and equipment upgrades.
- The potential energy savings and benefits must be carefully weighed against the upfront and ongoing costs to ensure economic viability.
Despite these limitations, ongoing research, technological advancements, and collaboration among industry experts and research institutions can help overcome some of these challenges. Continued innovation in materials, design techniques, control systems, and manufacturing processes can lead to improvements in steam turbine performance while maintaining reliability, availability, safety factors, and environmental compliance.
WHY, WHEN, WHERE, WHAT, WHICH, HOW TO APPLY IMPROVEMENTS IN STEAM RATE, POWER DENSITY & THERMAL EFFICINECY IN SPECIAL STEAM TURBINES
Why:
- Improving the steam rate, thermal efficiency, and power density helps optimize energy conversion and reduces fuel consumption, leading to cost savings and reduced environmental impact.
- Enhancing reliability, availability, and safety factors ensures consistent operation, minimizes downtime, and mitigates potential risks and failures.
When:
- The optimization efforts should be initiated during the design phase of new projects or when planning major upgrades to existing steam turbine systems.
- Periodic assessments and optimization studies should also be conducted throughout the lifecycle of the turbines to identify potential improvements.
Where:
- Special purpose steam turbines are typically used in power generation, oil, and gas industries where high-pressure steam is generated, and efficient energy conversion is crucial.
- They are commonly employed in applications such as combined heat and power (CHP) plants, refineries, petrochemical facilities, and industrial cogeneration.
What:
- Improving the steam rate involves enhancing the mass flow rate of steam through the turbine, which can be achieved through the optimization of steam path components, such as nozzles, blades, seals, and diaphragms.
- Enhancing thermal efficiency focuses on minimizing energy losses by improving turbine design, maximizing steam conditions, optimizing clearances, and reducing parasitic losses from the casing and associated piping systems.
- Increasing power density entails increasing the power output for a given turbine size, which can be achieved through advanced blade designs, higher steam temperatures and pressures, and improved aerodynamics.
Which:
- Identify the specific areas within the steam turbine system that have the most potential for improvement, such as blade profiles, nozzle designs, sealing mechanisms, cooling systems, and control strategies.
- Determine the appropriate technology upgrades or retrofits that align with the specific goals and constraints of the application, considering factors such as available space, compatibility, and economic viability.
How:
- Utilize advanced computational modeling and simulation techniques, such as computational fluid dynamics (CFD), finite element analysis (FEA), and thermodynamic modeling, to optimize the turbine design, analyze flow characteristics, and assess performance improvements.
- Conduct experimental studies, including component testing and validation, to verify the effectiveness of design modifications and technology upgrades.
- Implement advanced monitoring and control systems, including real-time condition monitoring, predictive maintenance, and performance optimization algorithms, to ensure efficient and reliable turbine operation.
- Collaborate with industry experts, research institutions, and equipment suppliers to share knowledge, leverage advancements in turbine technology, and foster innovation through research and development efforts.
By addressing the “why, when, where, what, which, and how” aspects outlined above, it is possible to implement strategies and technologies that improve the steam rate, thermal efficiency, and power density in special purpose steam turbines. This leads to enhanced reliability, availability, safety factors, and environmental compliance in power generation, oil, and gas industries.
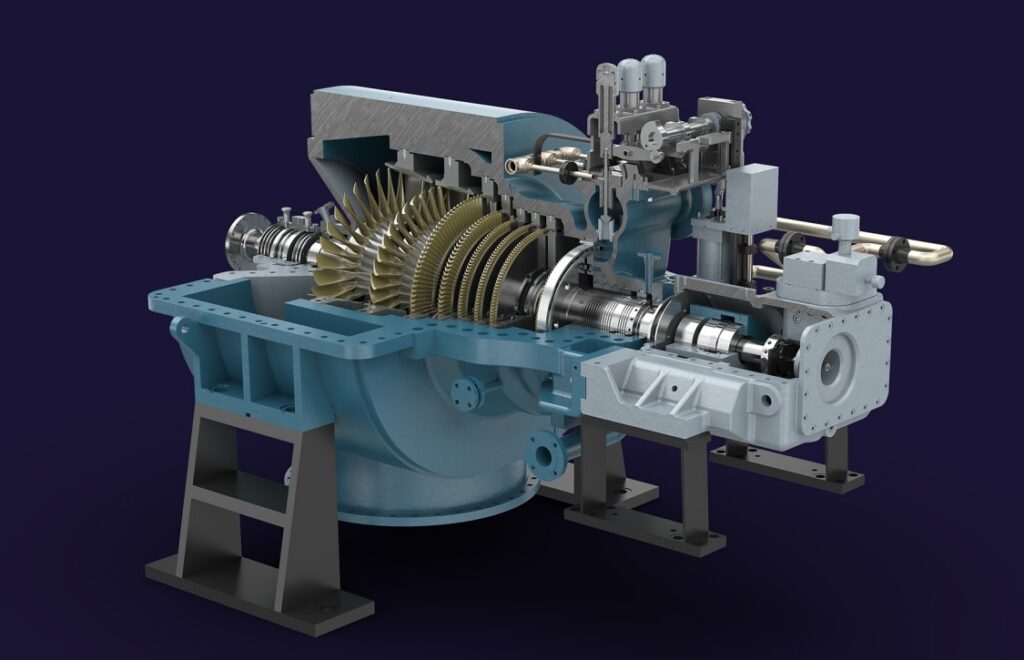
PROCEDURES, ACTIONS, STUDIES, MITIGATION, RECOMMENDATIONS TO IMPROVE STEAM RATE, POWER DENSITY & THERMAL EFFICIENCY IN SPECIAL STEAM TURBINES
Procedures and Actions: a. Conduct a comprehensive assessment of the existing steam turbine system, including performance evaluation, operational data analysis, and identification of bottlenecks or areas for improvement. b. Develop a detailed design plan that includes specific measures to optimize the steam rate, thermal efficiency, and power density. c. Implement advanced control strategies to enhance operational flexibility and optimize turbine performance under various operating conditions. d. Upgrade or retrofit key components, such as blades, nozzles, seals, and diaphragms, using advanced materials and designs that offer improved efficiency and durability. e. Enhance steam path cooling mechanisms to mitigate overheating and ensure efficient heat transfer. f. Implement advanced sealing technologies to minimize steam leakage and improve efficiency. g. Improve the aerodynamic profile of blades and nozzles to optimize steam flow and minimize energy losses. h. Optimize clearances between rotating and stationary components to reduce leakage and improve turbine efficiency. i. Enhance turbine rotor dynamics and balancing to minimize vibration and ensure reliable operation.
Studies and Mitigation Measures: a. Conduct computational modeling and simulation studies, such as CFD and FEA, to analyze flow characteristics, heat transfer, and structural behavior within the turbine system. b. Perform experimental studies, including component testing and validation, to verify the performance of design modifications and technology upgrades. c. Evaluate and mitigate potential failure modes, such as blade vibration, erosion, corrosion, and fatigue, through detailed risk assessments and appropriate design enhancements. d. Implement effective maintenance and inspection programs to identify and address issues proactively, including regular monitoring of turbine health and performance. e. Implement condition-based maintenance strategies that utilize real-time monitoring and data analytics to optimize maintenance intervals and reduce downtime.
Recommendations: a. Collaborate with turbine manufacturers, research institutions, and industry experts to leverage the latest advancements in turbine design, materials, and technologies. b. Invest in research and development efforts to explore innovative approaches to improve steam rate, thermal efficiency, and power density in steam turbines. c. Consider the potential benefits of combined cycle configurations, where waste heat from the steam turbine can be utilized to generate additional power or provide process heat. d. Implement comprehensive environmental management practices, including emissions control technologies, to minimize environmental impacts associated with steam turbine operations. e. Establish a robust training program for turbine operators and maintenance personnel to ensure proper operation, maintenance, and troubleshooting of the turbine system. f. Continuously monitor and evaluate performance metrics, such as steam rate, thermal efficiency, power output, and reliability indicators, to identify areas for further improvement.
By following these procedures, actions, conducting studies, implementing mitigation measures, and incorporating the recommended practices, it is possible to achieve significant improvements in the steam rate, thermal efficiency, and power density of special-purpose steam turbines. This leads to enhanced reliability, availability, safety factors, and reduced environmental risks and critical failures in power generation, oil, and gas industries.
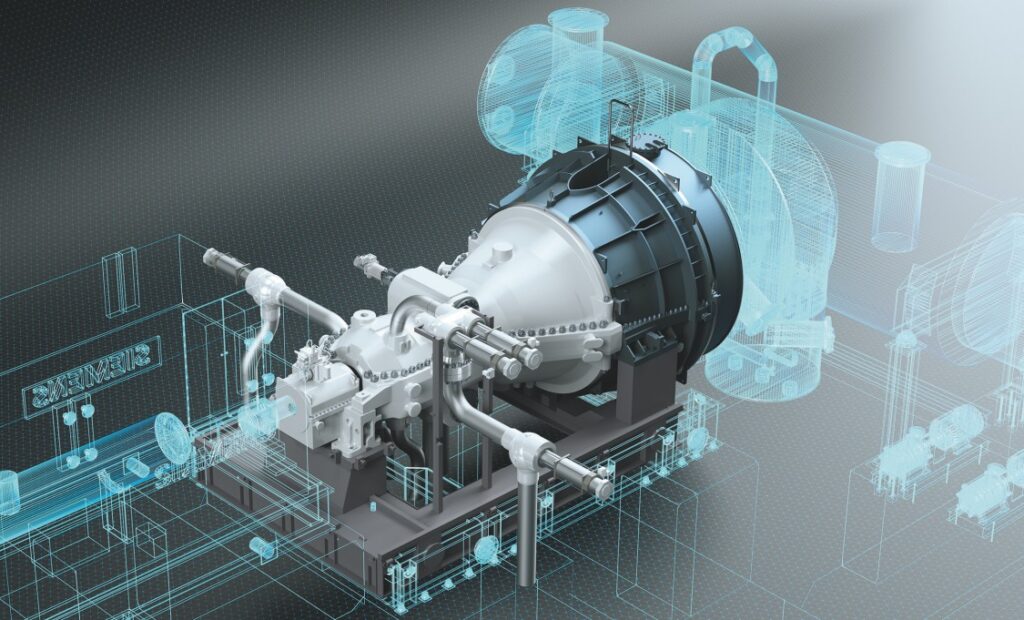