RECENT TECHNOLOGY IN MATERIALS FOR GAS TURBINES
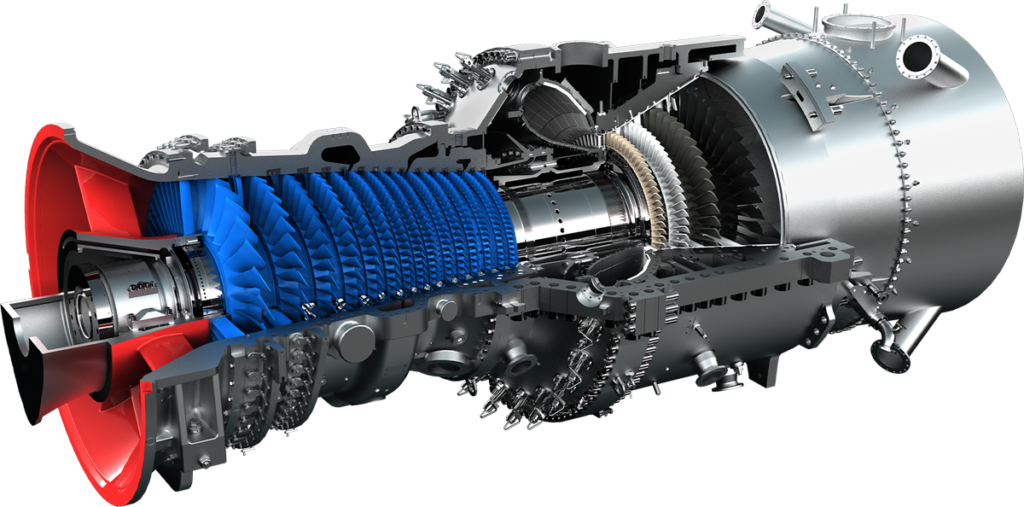
Gas turbines are critical components in the power generation and aviation industries. They rely on high-temperature materials such as alloys to withstand the extreme operating conditions. However, these materials are susceptible to corrosion, erosion, and oxidation, which can lead to significant performance degradation, safety hazards, and costly downtime.
To address these challenges, researchers have developed various coating barriers, ceramics, and other advanced materials that can enhance the performance and reliability of gas turbine components. These materials are designed to improve efficiency, reduce emissions, and extend the life of critical parts such as turbine and compressor blades, wheels, combustors, transition pieces, and exhaust sections.
One approach to improving the durability of gas turbine components is through the use of thermal barrier coatings (TBCs). TBCs are typically made from ceramic materials that can withstand high temperatures and thermal stresses. They are applied to the surface of the component to create a barrier between the hot gases and the underlying metal. This reduces heat transfer to the metal, which helps to reduce thermal fatigue, oxidation, and corrosion.
Another strategy is to use advanced alloys that offer better high-temperature performance and resistance to corrosion and fatigue. For example, nickel-based superalloys are commonly used in gas turbine components due to their excellent high-temperature strength and resistance to creep, corrosion, and oxidation. Additionally, some alloys are designed to have a low coefficient of thermal expansion, which can help to reduce thermal stresses and extend the life of the component.
In addition to materials selection, researchers are also exploring advanced manufacturing techniques to improve the performance and reliability of gas turbine components. For example, additive manufacturing (AM) can be used to produce complex geometries with improved performance and reduced weight. AM also allows for the production of customized components that are tailored to specific operating conditions and requirements.
Overall, ongoing research and development in the field of materials science and engineering are critical for improving the efficiency, reliability, safety, and performance of gas turbine components. By developing advanced materials and manufacturing techniques, researchers can help to reduce costs, improve performance, and extend the life of gas turbines in both new projects and existing plants.
LIMITS IN ENGINEERING & DESIGN IN MATERIALS
Despite ongoing research and development in the field of materials science and engineering, there are still some limits in engineering and design when it comes to critical materials used in gas turbines.
One of the key challenges is finding materials that can withstand the extreme operating conditions in gas turbines. These conditions include high temperatures, thermal cycling, corrosion, erosion, and mechanical stresses. While some materials are designed to perform well under certain conditions, it can be difficult to find materials that can meet all of the requirements for a specific gas turbine application.
In addition to material selection, there are also limitations in the design of gas turbine components. For example, it can be difficult to design components with complex geometries that can withstand the high stresses and temperatures in gas turbines. This is particularly challenging for components that require cooling, such as turbine blades and vanes. Designing effective cooling schemes that can keep the components within a safe operating temperature range while also maintaining structural integrity is a complex task that requires careful consideration of many factors.
Another limitation in engineering and design is the cost of developing and testing new materials and components. The development of new alloys, thermal barrier coatings, and ceramics requires significant investment in research and development, testing, and certification. This can be a barrier for smaller companies or organizations that may not have the resources to invest in these activities.
Finally, there are also limitations in the manufacturing processes used to produce gas turbine components. Some manufacturing techniques, such as casting and forging, can result in components with defects that can lead to premature failure. It can also be difficult to achieve the necessary dimensional accuracy and surface finish required for gas turbine components. Advances in additive manufacturing have shown promise in overcoming some of these limitations, but further research is needed to fully understand the capabilities and limitations of these techniques.
Overall, while there have been significant advances in the development of critical materials used in gas turbines, there are still limitations in engineering and design that must be addressed to further improve the efficiency, reliability, safety, and performance of these components. Addressing these limitations requires ongoing research and development, as well as collaboration between materials scientists, engineers, and manufacturers.
WHY, WHEN, WHICH & WHERE TO APPLY ABOUT CRITICAL MATERIALS
WHY: The use of critical materials such as new alloys, thermal coating barriers, and ceramics in gas turbines is essential for improving their efficiency, performance, and reliability. Gas turbines operate in extreme conditions that can cause significant wear and damage to components, leading to reduced efficiency, higher maintenance costs, and increased risk of failures. Critical materials are designed to withstand these conditions and protect components from wear, corrosion, and thermal stresses, thus reducing downtime and increasing the lifespan of the gas turbine.
WHEN: Critical materials should be applied during the design and construction phase of gas turbine projects. This includes both new projects and upgrades to existing plants. During the design phase, engineers should carefully consider the operating conditions and requirements of the gas turbine and select materials that are suitable for the specific application. During construction, components should be manufactured with the selected materials and coatings to ensure their optimal performance.
WHICH: The selection of critical materials will depend on the specific application of the gas turbine. For example, for high-temperature applications, nickel-based superalloys are commonly used due to their excellent high-temperature strength and resistance to corrosion and oxidation. Thermal barrier coatings made from ceramics can also be used to reduce heat transfer and improve the lifespan of components. For components that require cooling, such as turbine blades, special alloys with good thermal conductivity can be used to improve heat dissipation.
WHERE: Critical materials should be applied in all critical components of gas turbines, including turbine and compressor blades, wheels, combustors, transition pieces, and exhaust sections. These components are subject to extreme operating conditions and require materials that can withstand high temperatures, corrosion, and mechanical stresses. The selection of materials should be carefully considered for each component based on its specific requirements and operating conditions. In addition, coatings such as thermal barrier coatings should be applied to the surface of components to protect them from wear and damage.
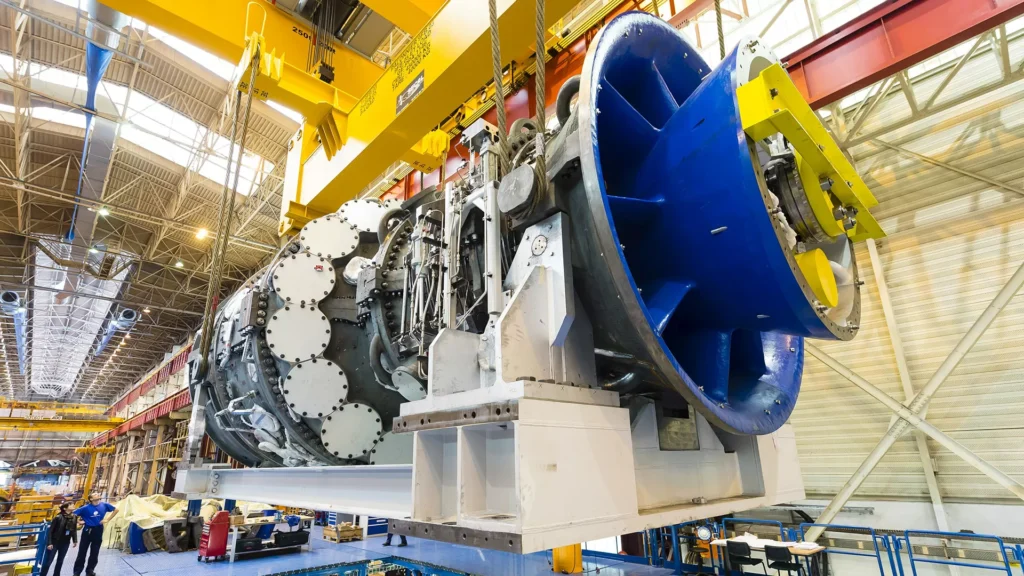
PROCEDURES, ACTIONS, STUDIES, MITIGATION, RECOMMENDATIONS USING NEW MATERIALS
To ensure the successful application of critical materials such as new alloys, thermal coating barriers, and new ceramics in gas turbines, the following procedures, actions, studies, and recommendations should be applied:
Conduct a thorough risk assessment: Before selecting critical materials, it’s essential to conduct a risk assessment to identify potential failure modes, causes, and consequences. The risk assessment should consider both the design and operating conditions of the gas turbine.
Select the appropriate materials: Based on the risk assessment, select the appropriate critical materials for each component of the gas turbine. The selection should be based on their ability to withstand the specific operating conditions and requirements of each component.
Conduct material testing and validation: Critical materials should be thoroughly tested and validated to ensure that they meet the required standards and specifications. This testing should include mechanical, thermal, and chemical testing to ensure that the materials can withstand the extreme conditions of gas turbine operation.
Apply thermal barrier coatings: For high-temperature applications, thermal barrier coatings should be applied to the surface of components to protect them from thermal stresses and improve their lifespan.
Monitor and maintain components: Once critical materials have been applied, regular monitoring and maintenance should be conducted to ensure that components are performing as expected. This monitoring should include regular inspections, vibration analysis, and thermal imaging.
Conduct failure analysis: In the event of a failure, a thorough failure analysis should be conducted to identify the root cause and develop corrective actions. This analysis should include both physical and metallurgical analysis of failed components.
Implement corrective actions: Based on the findings of the failure analysis, implement corrective actions to prevent similar failures in the future. These corrective actions may include changes to the design, selection of different materials, or changes to the maintenance procedures.
Continuous improvement: Finally, continuous improvement should be implemented to ensure that critical materials and components are continually optimized for improved efficiency, performance, reliability, and safety.
By following these procedures, actions, studies, and recommendations, the successful application of critical materials in gas turbines can be achieved, improving efficiency and performance while reducing the risk of critical failures in new projects and existing plants in power generation and oil and gas industries.
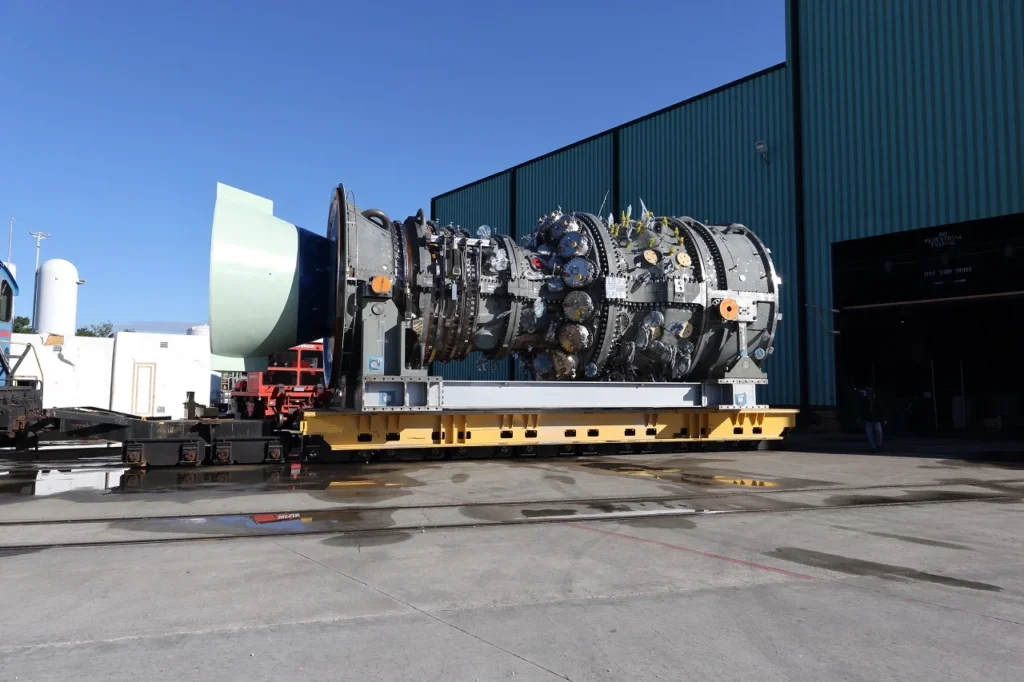