ADVANCES IN TECHNOLOGY - SPECIAL STEAM TURBINES
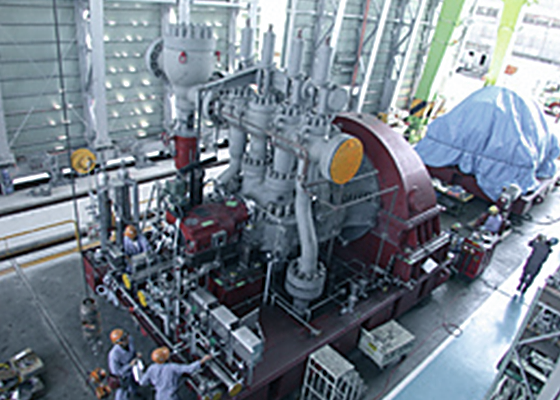
DIFFERENCES - IMPULSE vs REACTION - SPECIAL STEAM TURBINES - APPLICABILITY
ROTODYNAMIC TECHNOLOGY FOR SPECIAL STEAM TURBINES
Reaction and impulse steam turbines are two types of steam turbines that have distinct design characteristics and are used for different applications in various industries, including power generation and oil and gas.
Impulse steam turbines are typically used in power generation plants and are designed to handle high-pressure and low-flow steam conditions. In an impulse turbine, the steam flows through a series of nozzles, which convert its pressure into kinetic energy, and then into mechanical energy through the turbine blades. The rotor of an impulse turbine is supported by bearings located at each end of the shaft.
Reaction steam turbines, on the other hand, are typically used in industries such as oil and gas, chemical, and petrochemical, and are designed to handle high-flow and low-pressure steam conditions. In a reaction turbine, the steam flows through a series of stationary blades (stator) and then through a series of rotating blades (rotor), which convert the steam’s pressure and velocity into mechanical energy. The rotor of a reaction turbine is typically supported by journal bearings, which allow for axial movement of the rotor.
In terms of applicability in power generation plants, impulse turbines are typically used in low-pressure and high-flow conditions, such as in a nuclear or fossil fuel power plant. Reaction turbines are used in high-pressure and low-flow conditions, such as in a hydroelectric power plant or in a combined cycle power plant. Reaction turbines are also commonly used in the oil and gas industry for power generation or to drive compressors or pumps.
In terms of environmental concerns, both impulse and reaction turbines can contribute to greenhouse gas emissions, as they typically rely on fossil fuels to generate the steam required for operation. However, modern designs and technologies, such as the use of renewable energy sources or carbon capture and storage, can help reduce the environmental impact of these turbines.
In terms of reliability, availability, and safety, both types of turbines must be designed and maintained to meet the specific requirements of their applications. Proper maintenance, including regular inspection and replacement of worn or damaged parts, is essential to ensure the safe and reliable operation of both impulse and reaction turbines. Additionally, appropriate safety measures must be in place to prevent critical failures and unscheduled shutdowns, which can result in equipment damage, injury, or even loss of life.
Overall, the choice between impulse and reaction turbines depends on the specific requirements of the application, including steam conditions, power output, and environmental considerations. Both types of turbines have their advantages and limitations, and proper engineering and design are critical to ensure their reliable and safe operation in power generation plants and in the oil and gas industry.
Rotodynamic technology is a field of engineering that deals with the analysis and design of rotating machinery such as turbines, compressors, and pumps. In the context of special steam turbines, rotodynamic technology plays a crucial role in improving their reliability and safety.
One of the key aspects of rotodynamic technology in special steam turbines is lateral analysis, which involves studying the lateral vibrations of the turbine shaft and bearings. This is important because excessive vibration can lead to mechanical failure and reduced turbine efficiency. Lateral analysis helps in identifying the critical speeds and modes of vibration that could potentially cause problems and allows for the selection of appropriate bearings and dampers to mitigate the risk.
Torsional analysis is another important aspect of rotodynamic technology in special steam turbines. It involves analyzing the torsional vibrations of the turbine shaft and is crucial in ensuring that the shaft remains stable and does not break due to fatigue. Torsional analysis helps in identifying the natural frequencies of the shaft and enables the design of appropriate torsional damping systems.
Stiffness-stability maps are also used in rotodynamic technology to optimize the design of special steam turbines. These maps are graphical representations of the relationship between the stiffness and stability of the rotor system. They help in identifying the most stable operating conditions for the turbine and enable the selection of appropriate bearing and damper configurations.
Resonance and structural issues are other important considerations in the design of special steam turbines. Resonance can occur when the natural frequency of the rotor system coincides with the operating frequency of the turbine, leading to excessive vibration and potential failure. Structural issues such as fatigue and cracking can also occur due to the cyclic loading of the turbine components. Mitigation measures such as careful material selection, appropriate design of the turbine components, and regular maintenance can help in avoiding such issues.
Overall, rotodynamic technology plays a critical role in improving the reliability, safety, and efficiency of special steam turbines in power generation plants and in the oil and gas industry.
LIMITS IN ENGINEERING & DESIGN - COMPARATIVE IMPULSE vs REACTION TYPE
LIMITS IN ENGINEERING & DESIGN OF ROTODYNAMIC TECHNOLOGY IN SPECIAL STEAM TURBINES
The selection of a steam turbine type for a specific application depends on various factors, including the characteristics of the steam, operating conditions, load requirements, and other specific needs of the application.
In terms of engineering and design limits, reaction turbines tend to be more complex and costly than impulse turbines due to the need for multiple stages and more precise tolerances. They are also more susceptible to vibration and require more frequent maintenance. Impulse turbines, on the other hand, have simpler designs and are less expensive to manufacture, but are less efficient at low-pressure ratios and cannot handle as much steam flow.
When it comes to power generation plants, both reaction and impulse turbines have their advantages and disadvantages. Reaction turbines are well-suited for large-scale power generation applications where high efficiency is critical, while impulse turbines are typically used for small-scale power generation applications or where low-pressure steam is available.
In the oil and gas industry, steam turbines are commonly used for applications such as oil and gas processing, pipeline compression, and steam injection for enhanced oil recovery. The choice of turbine type will depend on the specific needs of the application. Reaction turbines may be preferred for high-pressure steam applications such as pipeline compression, while impulse turbines may be more suitable for low-pressure steam applications.
Overall, the engineering and design limits of steam turbines, whether reaction or impulse type, depend on the specific needs of the application and the operating conditions. Proper selection, installation, and maintenance are critical to ensuring high reliability, availability, safety, and environmental performance while avoiding critical failures and unscheduled shutdowns.
The limits in engineering and design of rotodynamic technology being applicable in special steam turbines are important to consider in order to ensure high reliability, availability, safety, and avoid critical and environmental failures and unscheduled shutdowns in power generation plants and oil & gas industries.
One limit is the accuracy of the lateral analysis, which is used to determine the critical speeds and modes of the rotor system. If the lateral analysis is inaccurate, the rotor system may experience excessive vibrations, which can lead to premature failure of bearings and other components. Therefore, it is important to use accurate measurement and simulation techniques to ensure that the lateral analysis is reliable.
Another limit is the accuracy of the torsional analysis, which is used to determine the natural frequency and mode shapes of the rotor system in torsion. If the torsional analysis is inaccurate, the rotor system may experience torsional vibrations, which can lead to fatigue failure of shafts and other components. Therefore, it is important to use accurate measurement and simulation techniques to ensure that the torsional analysis is reliable.
The stiffness-stability map is another important consideration in the design of special steam turbines. This map shows the relationship between the stiffness of the rotor system and its stability, and helps to determine the optimal stiffness values for different operating conditions. If the stiffness-stability map is not properly designed, the rotor system may experience instability, which can lead to catastrophic failure of the turbine. Therefore, it is important to carefully design the stiffness-stability map based on accurate measurement and simulation techniques.
Resonance mechanical and structural issues are also important limits to consider in the design of special steam turbines. These issues can arise when the natural frequencies of the rotor system and the support structure are in resonance, leading to excessive vibrations and potential failures. Therefore, it is important to carefully design the rotor system and the support structure to avoid resonance conditions and ensure reliable operation.
In summary, the limits in engineering and design of rotodynamic technology being applicable in special steam turbines involve ensuring the accuracy of lateral and torsional analysis, designing an optimal stiffness-stability map, and avoiding resonance mechanical and structural issues. By carefully considering these limits, it is possible to improve the reliability and safety of special steam turbines in power generation plants and oil & gas industries.
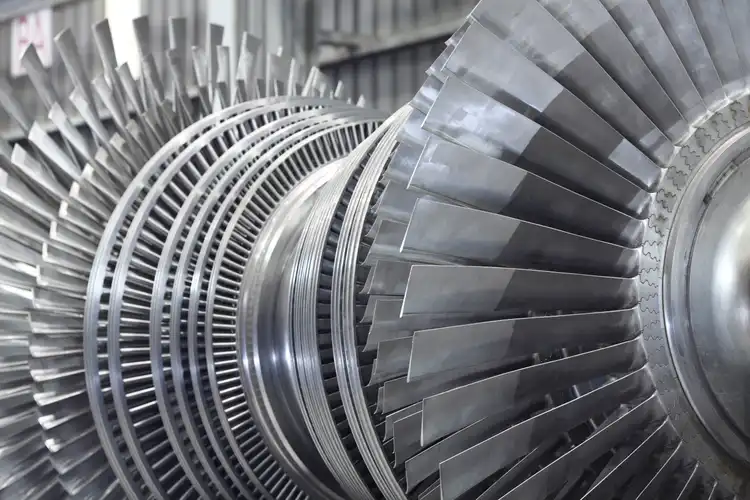
CRITICAL RISKS AND FAILURES, AND PROCEDURES, ACTIONS, STUDIES, RECOMENDATIONS TO USE IMPULSE vs REACTION TYPE DESIGN
CRITICAL RISKS & FAILURES, PROCEDURES, ACTIONS, STUDIES, RECOMMENDATIONS IN ROTODYNAMIC TECHNOLOGY
The critical risks and failures associated with the use of special steam turbines, whether reaction or impulse type, in power generation plants and oil and gas industries include:
Wear and tear: As with any machinery, steam turbines are subject to wear and tear, which can lead to component failures, power loss, and unscheduled downtime.
Vibration: High levels of vibration can cause mechanical failures, such as fatigue and cracks in components, and can also cause damage to bearings and other parts of the turbine.
Corrosion: Steam turbines are often exposed to harsh environments and high temperatures, which can cause corrosion and erosion of components.
Water quality: The quality of the water used in the steam cycle can affect the reliability and efficiency of the turbine. Impurities in the water can cause corrosion and scaling, leading to reduced efficiency and power output.
To mitigate these risks and failures, procedures and actions can be taken such as:
Regular maintenance: Regular maintenance, including inspections, cleaning, and replacement of worn or damaged parts, can help to prevent failures and prolong the life of the turbine.
Monitoring: Regular monitoring of vibration levels, temperature, and other parameters can help to detect potential issues before they become critical.
Water treatment: Proper water treatment, including filtration and chemical treatment, can help to prevent scaling, corrosion, and other water-related issues.
Upgrades and modifications: Upgrading and modifying the turbine, such as adding sensors or improving the control system, can improve reliability and efficiency.
Training and education: Providing training and education to the staff operating and maintaining the turbine can improve safety and reduce the risk of human error.
The limits in engineering and design when choosing between reaction and impulse type steam turbines depend on the specific application and operating conditions. For example, reaction turbines are better suited for high-pressure, high-flow applications, while impulse turbines are better suited for low-pressure, low-flow applications. The choice of turbine also depends on factors such as efficiency, cost, and maintenance requirements. It is important to carefully consider these factors when selecting a steam turbine for a particular application.
The critical risks and failures associated with the rotodynamic technology being applicable in special steam turbines mainly relate to mechanical and structural issues that can result in the failure of the turbine, unscheduled shutdowns, and potentially catastrophic accidents. Some of these risks and failures include:
- Lateral instability leading to vibration, which can cause damage to the turbine and other components in the system.
- Torsional instability leading to excessive twisting and potential damage to the turbine.
- Resonance, which can cause significant vibration and damage to the turbine and surrounding equipment.
- Structural issues, including material fatigue and creep, which can lead to catastrophic failures.
To mitigate these risks, procedures and actions need to be taken, including the following:
- Conducting thorough lateral and torsional analyses to ensure the turbine’s stability and avoid vibration issues.
- Developing stiffness-stability maps to ensure the turbine’s structural integrity and avoid resonance issues.
- Using advanced design and analysis tools to identify and address any potential mechanical and structural issues.
- Conducting regular inspections and maintenance to monitor the condition of the turbine and identify any potential issues before they become critical.
- Implementing appropriate safety protocols and emergency response plans to minimize the risk of accidents.
In addition to these mitigation measures, it is also essential to ensure that the turbine’s design is appropriate for the intended application and operating conditions. The limits in engineering and design need to be carefully considered, and any potential issues addressed during the design phase to ensure the turbine’s reliability, availability, and safety.
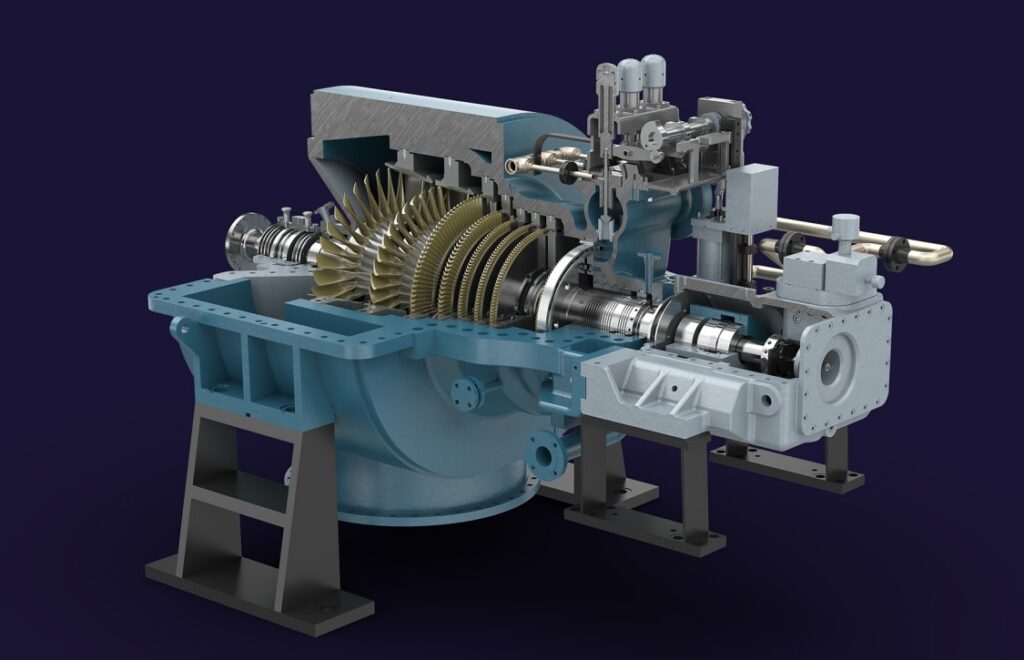
RERATES, UPGRADES, MODIFICATIONS IN DESIGN OF SPECIAL STEAM TURBINES
REFURBISHMENT TECHNIQUES FOR STATIONARY COMPONENTS - SPECIAL STEAM TURBINES
Rerates, upgrades, and modifications for special steam turbines are common practices in the power generation and oil & gas industries. These actions are taken to improve the reliability, safety, maintainability, and availability of the turbines, and to avoid critical and degradation failures and unscheduled shutdowns.
Rerates involve increasing the power output of the turbine without increasing its size or changing its operating conditions. This can be done by optimizing the design of the blades and other components, increasing the steam flow rate, or using advanced materials. Rerates can be a cost-effective way to increase power output and extend the lifespan of the turbine.
Upgrades involve replacing or modifying existing components with newer, more advanced ones. This can include upgrading the control system, replacing worn or damaged parts, or improving the cooling system. Upgrades can improve the efficiency and performance of the turbine, as well as extend its lifespan.
Modifications involve making changes to the design or operation of the turbine to address specific issues or improve its overall performance. This can include modifying the blade geometry to reduce vibration or increasing the clearance between the blades and casing to reduce the risk of rubbing. Modifications can be particularly useful for addressing issues that arise during operation or for adapting the turbine to changing operational requirements.
To ensure the success of rerates, upgrades, and modifications, a thorough understanding of the turbine’s design and operating conditions is necessary. This requires detailed engineering analysis, including stress and fatigue analysis, computational fluid dynamics (CFD) analysis, and thermal analysis. Any modifications or upgrades must be carefully tested and validated to ensure they do not negatively impact the turbine’s performance or reliability.
In conclusion, rerates, upgrades, and modifications are important tools for improving the reliability, safety, maintainability, and availability of special steam turbines in power generation plants and oil & gas industries. These actions must be carefully planned and executed, and validated through thorough analysis and testing, to ensure their success.
Refurbishment techniques for stationary components in special steam turbines can help improve their reliability, safety, maintainability, and availability in power generation plants and oil & gas industries. The refurbishment process generally involves the repair or replacement of components that have suffered wear or damage, as well as the modification of the original design to improve its performance and efficiency.
Some common refurbishment techniques for stationary components include:
Welding repair: This technique involves welding worn or damaged components to restore their original shape and function. The welding process may also involve the use of specialized coatings to improve the component’s resistance to wear and corrosion.
Thermal spraying: This technique involves the application of a high-temperature coating to the surface of components to improve their wear resistance and durability. Thermal spraying can be used to repair or modify a wide range of components, including rotors, casings, and blades.
Re-blading: This technique involves the replacement of worn or damaged blades in the turbine rotor. Re-blading may involve the use of new or refurbished blades, and can be used to improve the turbine’s efficiency and power output.
Balancing: This technique involves the adjustment of the rotor’s balance to reduce vibration and improve its reliability. Balancing may involve the addition or removal of material from the rotor or the use of specialized coatings to improve its balance.
Upgrades: This technique involves the modification of the original design to improve its performance, efficiency, and reliability. Upgrades may involve the use of new materials, coatings, or designs to improve the component’s resistance to wear, corrosion, or fatigue.
The refurbishment process should be performed by qualified and experienced technicians and should be based on a thorough assessment of the component’s condition and performance. Regular maintenance and inspection can help identify potential problems and allow for timely refurbishment to avoid critical failures and unscheduled shutdowns.
LIMITS IN ENGINEERING & DESIGN TO APPLY THE RERATES, UPGRADES & MODIFICATIONS
LIMITS IN ENGINEERING & DESIGN FOR REFURBISHMENT TECHNIQUES IN STATIONARY COMPONENTS
When applying rerates, upgrades, and modifications to special steam turbines, there are several limits in engineering and design that must be considered. These include:
Design limitations: The original design of the steam turbine may have certain limitations in terms of materials used, operating conditions, and power output. These limitations must be carefully considered when planning modifications to ensure that the upgraded turbine will still be safe and reliable.
Compatibility with existing systems: The modified steam turbine must be compatible with the existing systems, including piping, electrical systems, and control systems. Changes to the turbine may require modifications to these systems, which can be costly and time-consuming.
Regulatory compliance: Any modifications to the steam turbine must comply with relevant regulations and standards, such as safety codes and environmental regulations. Failure to comply with these regulations can result in penalties and legal liability.
Cost-effectiveness: The cost of rerates, upgrades, and modifications must be balanced against the benefits of improved reliability, safety, maintainability, and availability. The cost of the modifications should not outweigh the potential benefits.
Availability of spare parts: Modifications to the steam turbine may require replacement parts that are not readily available. This can increase maintenance costs and lead to longer downtimes if spare parts are not readily available.
Impact on other equipment: Modifications to the steam turbine may have an impact on other equipment within the plant. This impact must be carefully considered to ensure that the modifications do not cause problems with other equipment or systems.
Future operability: Any modifications must take into account the long-term operability of the steam turbine. Modifications should be designed to extend the lifespan of the turbine and ensure that it can continue to operate reliably for years to come.
Overall, it is essential to carefully evaluate the limits in engineering and design before applying rerates, upgrades, and modifications to special steam turbines. This will ensure that the modifications are safe, reliable, and cost-effective and do not cause problems with other systems or equipment within the plant.
The refurbishment techniques for stationary components in special steam turbines can help to improve the reliability, safety, maintainability, and availability of power generation plants and oil and gas industries. However, there are certain limits in engineering and design that need to be considered when applying these techniques.
One of the primary limits is related to the age and condition of the equipment. If the equipment is too old or damaged beyond repair, it may not be feasible to refurbish the stationary components. Additionally, the original design of the equipment may not be compatible with refurbishment techniques, requiring modifications that could compromise its performance or safety.
Another limit is related to the materials used in the stationary components. Refurbishment techniques may not be effective if the materials are prone to fatigue or corrosion. In some cases, it may be necessary to replace the materials altogether, which could be expensive and time-consuming.
Finally, refurbishment techniques must be carefully planned and executed to avoid introducing new failures or issues into the equipment. The refurbishment process should include thorough testing and analysis to ensure that the stationary components meet the required performance and safety standards.
Therefore, it is important to consult with experienced engineers and technicians in the field of steam turbines and to follow industry best practices to ensure that the refurbishment techniques are applied effectively and safely within the limits of engineering and design.
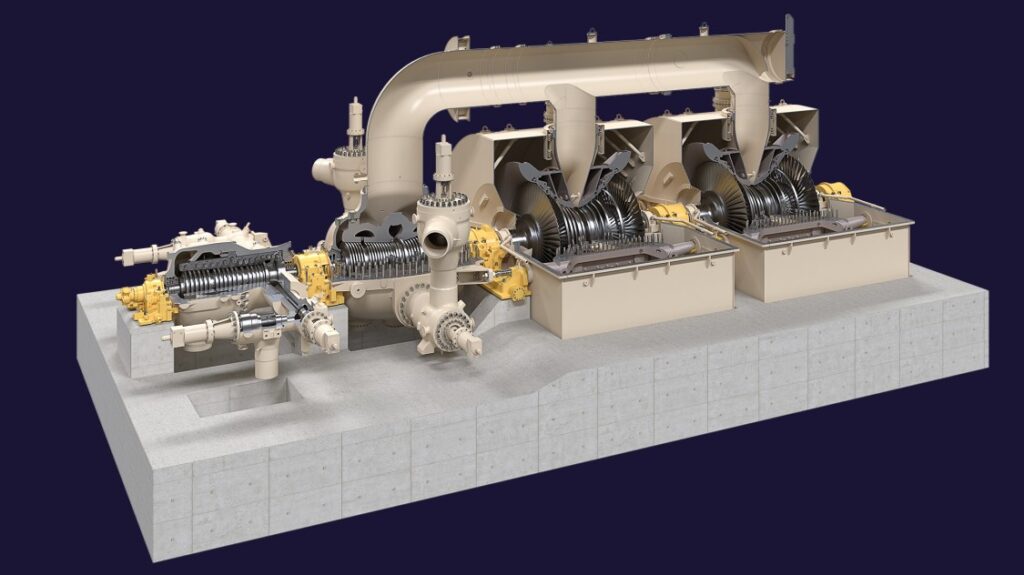
CRITICAL RISKS & FAILURES, PROCEDURES, ACTIONS, STUDIES, RECOMMENDATIONS FOR RERATES, UPGRADES & MODIFICATIONS
CRITICAL RISKS & FAILURES, PROCEDURES, ACTIONS, STUDIES, RECOMMENDATIONS FOR REFURBISHMENT TECHNIQUES IN STATIONARY PARTS IN SPECIAL STEAM TURBINES
When rerating, upgrading or modifying special steam turbines in power generation plants or oil and gas industries, there are several critical risks and failures that need to be considered. These include:
Compatibility issues: The new components or modifications must be compatible with the existing turbine system, including controls, instrumentation, and other equipment.
Design and engineering errors: The new components or modifications must be carefully designed and engineered to avoid any potential issues that may cause critical failures, such as increased stress levels or vibration.
Manufacturing and installation errors: Care must be taken during the manufacturing and installation process to ensure that all components are correctly installed and aligned to prevent any issues that may cause critical failures.
Operation and maintenance errors: Proper training and procedures must be implemented to ensure that the turbine is operated and maintained correctly to avoid any potential issues that may cause critical failures.
To mitigate these risks and failures, several actions and procedures can be taken:
Thorough assessment and analysis of the system before any modifications or upgrades are made, including stress and vibration analysis, fluid dynamics, and thermal analysis.
Use of advanced technologies such as finite element analysis, computational fluid dynamics, and rotor dynamics analysis to ensure the reliability and safety of the system.
Use of high-quality materials and components during the manufacturing process to ensure the durability and reliability of the system.
Proper training and procedures for operators and maintenance personnel to ensure the correct operation and maintenance of the system.
Regular monitoring and inspections of the system to detect any potential issues or degradation and take corrective action before they become critical.
In conclusion, rerating, upgrading, or modifying special steam turbines in power generation plants or oil and gas industries can help to improve their reliability, safety, maintainability, and availability. However, it is essential to carefully consider the critical risks and failures and implement appropriate procedures, actions, studies, mitigation, and recommendations to ensure that the modifications do not cause critical or degradation failures and unscheduled shutdowns.
Refurbishment techniques for stationary components in special steam turbines can help to improve their reliability, safety, maintainability, and availability in power generation plants and oil & gas industries. However, there are also critical risks and failures that should be considered, as well as procedures, actions, studies, mitigation measures, and recommendations.
Some critical risks and failures that can occur during the refurbishment of stationary components in special steam turbines include improper installation, incorrect material selection, inadequate quality control, improper surface preparation, and corrosion. These issues can lead to mechanical failures, reduced efficiency, increased maintenance costs, and unscheduled shutdowns.
To mitigate these risks and failures, it is important to follow proper procedures and actions during the refurbishment process. This can include conducting thorough inspections and assessments of the components, developing a detailed refurbishment plan, selecting appropriate materials and coatings, and ensuring proper installation and testing.
Studying the causes of previous failures can also provide valuable insights for improving the refurbishment process. This can include analyzing the failure mode, conducting root cause analysis, and implementing design improvements or process changes.
Recommendations for successful refurbishment of stationary components in special steam turbines include establishing a quality assurance program, ensuring proper training and certification of personnel involved in the process, and developing clear documentation and tracking systems to ensure traceability and accountability.
It is also important to consider the limits in engineering and design when applying refurbishment techniques to stationary components in special steam turbines. This includes understanding the limits of material properties, the impact of thermal and mechanical stresses, and the potential for fatigue and corrosion over time. Proper design and engineering considerations can help to ensure the long-term reliability and safety of refurbished components.
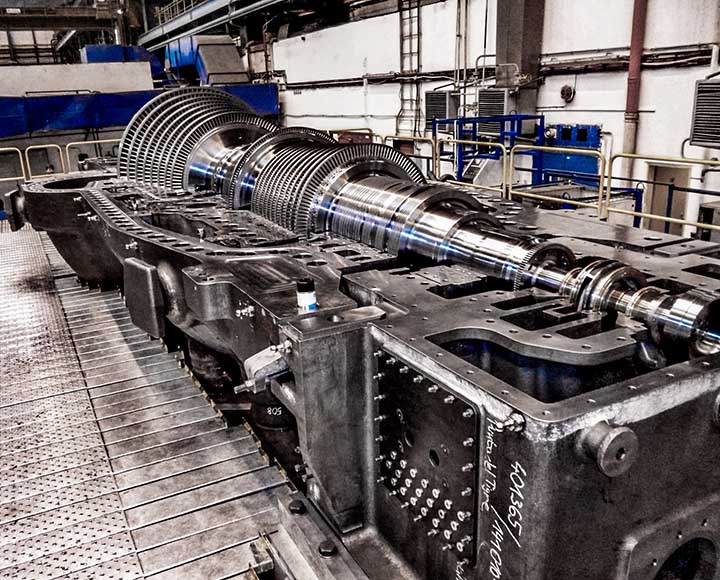