OREDA STUDIES (OFFSHORE & ONSHORE RELIABILITY DATA) - TURBOMACHINERY
CENTRIFUGAL COMPRESSORS
GAS TURBINES
SPECIAL STEAM TURBINES
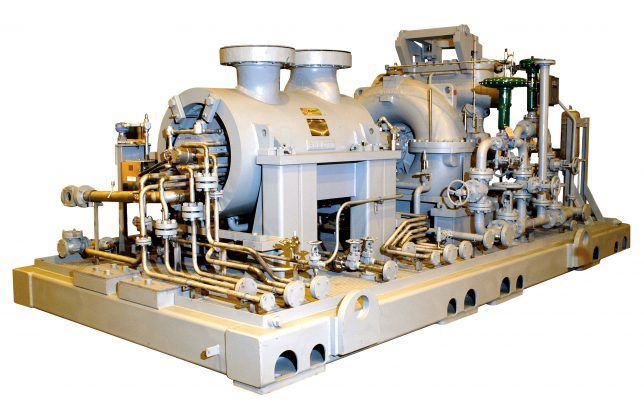
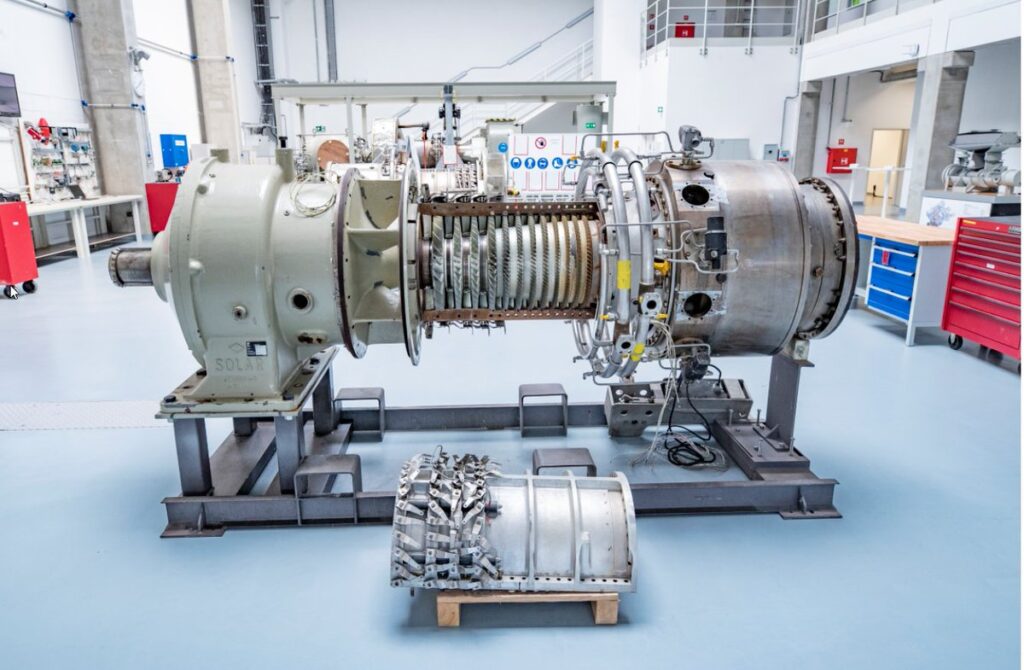
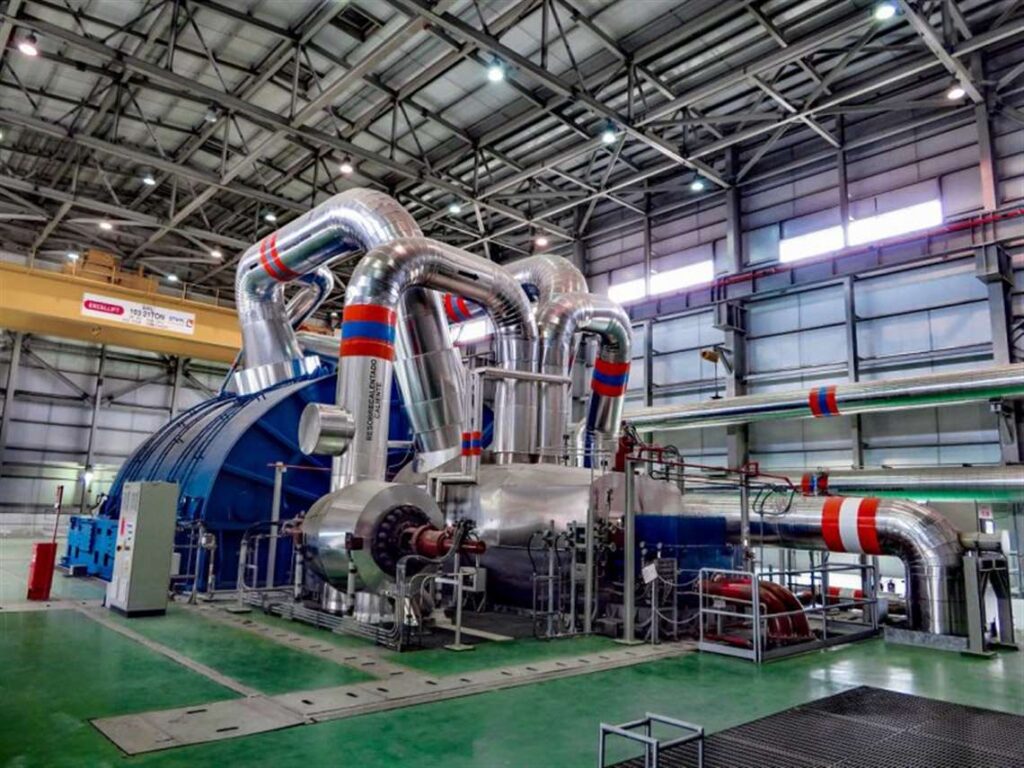
HISTORY ABOUT OREDA
LIMITATIONS IN DATA ADQUISITION
OREDA (Offshore/Onshore Reliability Data) was established in the late 1970s as a joint industry project aimed at collecting and sharing reliability data for offshore oil and gas facilities. The first edition of the OREDA handbook was published in 1981, and it contained reliability data for various types of equipment, including turbomachinery like centrifugal compressors, gas turbines, and steam turbines.
Since then, OREDA has undergone several phases of evolution. The second edition of the handbook was published in 1987, and it included data for additional equipment types, such as reciprocating compressors and pumps. In the 1990s, OREDA expanded its scope to include onshore facilities as well, and the third edition of the handbook was published in 1996.
In the early 2000s, OREDA began to focus on more advanced techniques for reliability data analysis, including Bayesian updating and fault tree analysis. The fourth edition of the handbook, published in 2002, included updated data for existing equipment types as well as new data for subsea equipment and offshore structures.
In the following years, OREDA continued to refine its methods and expand its scope, with the fifth edition of the handbook published in 2015. This edition included updated data for all equipment types, as well as new data for offshore wind turbines and other renewable energy systems.
Throughout its history, OREDA has focused on improving the reliability, maintainability, availability, and safety of equipment in the oil and gas industry. The data collected and analyzed by OREDA is used by equipment manufacturers, operators, and regulators to identify potential failure modes and develop strategies to mitigate those risks. The maintenance repair time data collected by OREDA is also used to inform maintenance scheduling and optimize maintenance practices, reducing downtime and improving overall equipment performance.
While OREDA (Offshore and Onshore Reliability Data) provides valuable information on the reliability and performance of machinery in the oil, gas, and petrochemical industries, there are several limitations to consider:
Participation: OREDA relies on participation from companies in the industry to collect data on equipment failures and maintenance practices. However, not all companies participate, which can result in gaps in the data and potentially biased results.
Data Quality: The reliability of the OREDA data is dependent on the quality and accuracy of the data provided by the participating companies. Incomplete or inaccurate data can lead to incorrect conclusions and recommendations.
Scope: The OREDA handbooks provide information on a wide range of equipment and failure modes, but they may not cover all possible failure modes or equipment types. For example, the handbooks may not cover newer equipment technologies or specialized equipment used in specific applications.
Applicability: While the OREDA handbooks provide guidance on the failure modes and maintenance practices associated with centrifugal compressors, the applicability of this information to specific compressor designs and operating conditions may vary.
Maintenance Time: OREDA provides valuable information on best practices for maintenance and monitoring of machinery, but the time required for maintenance can be a significant constraint in some operating environments. For example, offshore platforms may have limited access to equipment and require maintenance to be completed quickly and efficiently.
Despite these limitations, the information provided by OREDA can still be a valuable resource for improving the reliability, maintainability, availability, and safety of machinery in the oil, gas, and petrochemical industries. However, it is important to consider the limitations and apply the information judiciously in the context of specific equipment designs and operating conditions.
FAILURE TYPES
FAILURE MODES - TURBOMACHINERY
OREDA (Offshore and Onshore Reliability Data) categorizes failures into three types: critical, degradation, and incipient.
Critical Failures: These are failures that result in immediate and severe consequences, such as a catastrophic equipment failure or a safety incident. Critical failures can lead to significant downtime, production losses, and safety hazards.
Degradation Failures: These are failures that occur gradually over time, as equipment components wear out or degrade due to environmental factors or normal wear and tear. Degradation failures can lead to reduced equipment performance, increased maintenance requirements, and reduced asset life.
Incipient Failures: These are failures that have not yet caused equipment downtime or significant performance degradation, but which can potentially lead to more serious failures if left unaddressed. Incipient failures are often detected through condition monitoring techniques, such as vibration analysis, oil analysis, or visual inspections.
The OREDA handbooks provide information on the failure modes and mechanisms associated with each of these types of failures, as well as guidance on best practices for maintenance and monitoring to detect and prevent them. However, the time required for maintenance can be a significant constraint in some operating environments, and it is important to balance maintenance needs with operational requirements.
By understanding the different types of failures and their associated mechanisms, companies can prioritize their maintenance activities and optimize their maintenance schedules to reduce downtime, improve equipment reliability, and enhance safety in the oil, gas, and petrochemical industries.
The OREDA (Offshore and Onshore Reliability Data) handbooks provide information on the failure modes associated with centrifugal compressors, gas turbines, and steam turbines, as well as guidance on best practices for maintenance and monitoring to detect and prevent these failures. Some of the common failure modes for these types of machinery include:
Centrifugal Compressors: Failure modes for centrifugal compressors include bearing failures, seal failures, rotor imbalance, corrosion, and erosion. The OREDA handbooks provide detailed information on the frequency and severity of these failure modes, as well as guidance on best practices for maintenance and monitoring to prevent them.
Gas Turbines: Failure modes for gas turbines include blade and vane failures, combustor failures, bearing failures, and thermal fatigue. The OREDA handbooks provide information on the frequency and severity of these failure modes, as well as guidance on best practices for maintenance and monitoring to prevent them.
Steam Turbines: Failure modes for steam turbines include blade and vane failures, rotor and shaft failures, bearing failures, and thermal fatigue. The OREDA handbooks provide information on the frequency and severity of these failure modes, as well as guidance on best practices for maintenance and monitoring to prevent them.
In addition to these failure modes, the OREDA handbooks also provide information on other factors that can impact the reliability, maintainability, availability, and safety of machinery, such as environmental factors, operational conditions, and maintenance practices. By understanding the specific failure modes and risk factors associated with their machinery, companies can prioritize their maintenance activities and optimize their maintenance schedules to reduce downtime, improve equipment reliability, and enhance safety in the oil, gas, and petrochemical industries. However, it is important to consider the time required for maintenance and repair, and balance maintenance needs with operational requirements.
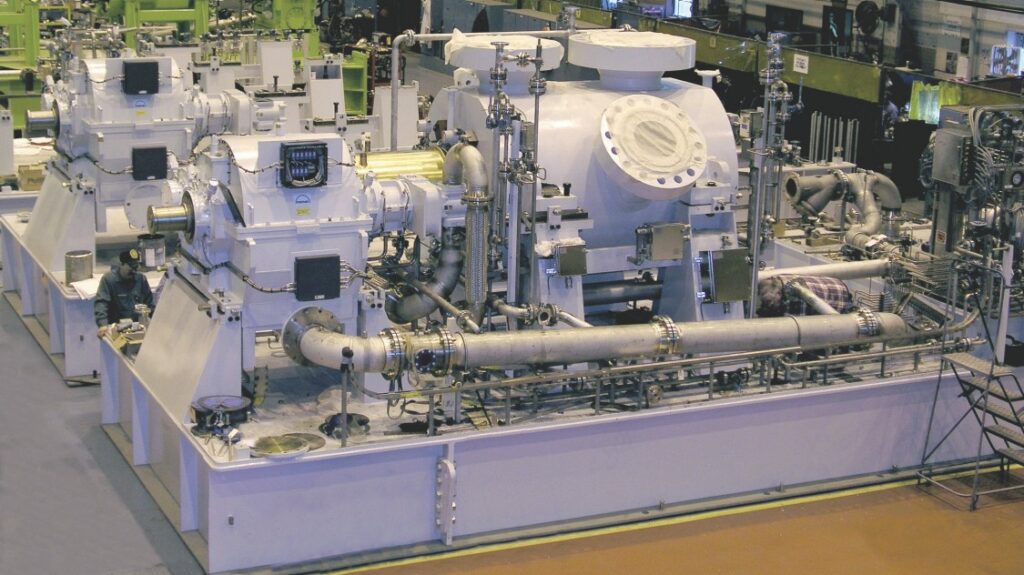
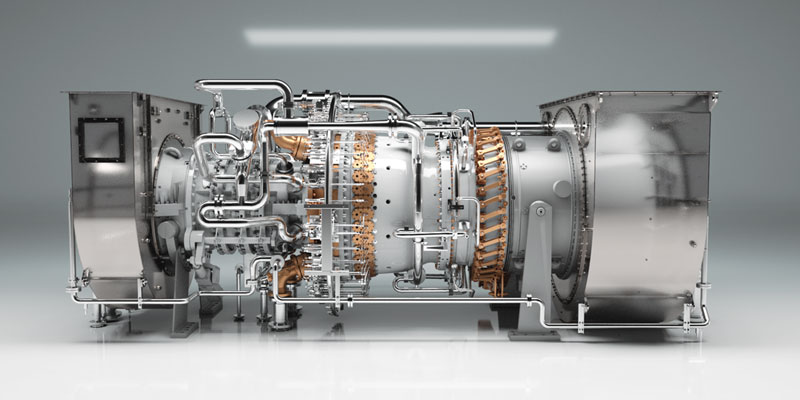
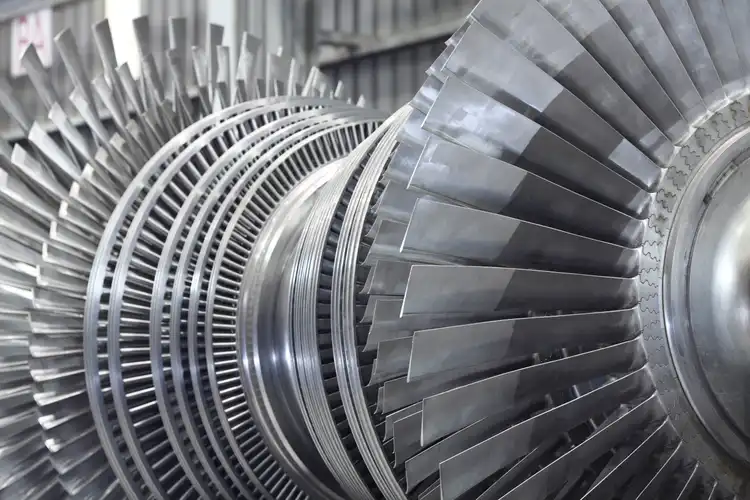
LIST OF FAILURE MODES USED BY OREDA
LIST OF FAILURE MECHANISMS USED BY OREDA
OREDA defines a comprehensive list of failure modes for turbomachinery, including:
Abnormal instrument reading: This refers to cases where instrument readings are outside the normal range, which could indicate an issue with the system being monitored. For example, abnormal temperature readings could indicate a problem with the cooling system.
Breakdown: This refers to a sudden and catastrophic failure of a component or system, resulting in a shutdown of the equipment. This can be caused by a variety of factors, including material failure, poor maintenance, or unexpected loads.
External leakages: This refers to leaks from external components, such as valves, pumps, or fittings. External leaks can lead to environmental damage, safety hazards, and loss of production.
Erratic output: This refers to situations where the output of the equipment varies unexpectedly or is outside of the desired range. This can be caused by issues with control systems, instrumentation, or other components.
Fail to start & to stop: This refers to issues with the equipment’s ability to start or stop when commanded. This can be caused by issues with control systems, fuel supply, or other components.
High & low output: This refers to situations where the output of the equipment is either too high or too low. This can be caused by issues with control systems, instrumentation, or other components.
Internal leakage: This refers to leaks from internal components, such as seals, bearings, or valves. Internal leaks can lead to reduced efficiency, increased wear and tear, and safety hazards.
Noise: This refers to excessive noise or vibration from the equipment, which can indicate issues with components such as bearings, seals, or rotors.
Overheating: This refers to situations where components or systems become too hot, which can cause damage to the equipment or create safety hazards.
Parameter deviation: This refers to cases where critical parameters such as temperature, pressure, or flow rate deviate from the desired range. This can be caused by issues with instrumentation, control systems, or other components.
Plugged/choked: This refers to situations where equipment components become plugged or choked with debris, which can lead to reduced efficiency, increased wear and tear, and safety hazards.
Minor in-service problems: This refers to issues that may not result in a shutdown or significant downtime, but can still lead to reduced efficiency, increased wear and tear, or safety hazards.
Structural deficiency: This refers to issues with the structural integrity of the equipment or supporting systems, such as cracks, corrosion, or fatigue.
Spurious stop: This refers to cases where the equipment shuts down unexpectedly, without a clear cause. This can be caused by issues with control systems, instrumentation, or other components.
Each of these failure modes can be evaluated using data from OREDA studies, and appropriate mitigation strategies can be developed to improve reliability, maintainability, availability, and safety in turbomachinery.
list of failure mechanisms used by OREDA and a brief explanation of each:
-
Blockage/Plugged: This refers to the obstruction of a flow path or channel, which could be caused by foreign objects, scale, or debris.
-
Breakage: This occurs when a component fractures or cracks under the load, and can be caused by material defects or excessive stress.
-
Burst: This refers to the sudden failure of a pressure-containing component, often due to overpressure or a material defect.
-
Clearance/Alignment Failure: This refers to the failure of components to maintain the correct clearance or alignment, often caused by wear or deformation.
-
Combined Causes: This refers to failures that are the result of multiple factors, such as a combination of wear and corrosion.
-
Contamination: This refers to the introduction of foreign substances into a component or system, which can cause damage or fouling.
-
Control Failure: This refers to the failure of control systems or components, which can lead to improper operation or damage to other components.
-
Corrosion: This refers to the degradation of materials due to chemical reactions with the environment, such as the corrosion of metal components in a corrosive gas stream.
-
Deformation: This refers to the change in shape or dimensions of a component or structure, which can be caused by excessive stress or temperature.
-
Electrical Failure: This refers to the failure of electrical components or systems, such as motors or control circuits.
-
External Influence: This refers to failures caused by external factors, such as weather events, operator error, or vandalism.
-
Faulty Power/Voltage: This refers to failures caused by issues with the power supply or voltage level, such as under-voltage or over-voltage conditions.
-
Faulty or No Signal/Indication/Alarm: This refers to failures caused by issues with the sensing, indication, or alarm systems, which can lead to improper operation or damage to other components.
-
Instrument Failure: This refers to the failure of instruments used for measurement or control, such as pressure or temperature sensors.
-
Leakage: This refers to the escape of fluids or gases from a component or system, which can lead to reduced performance or damage to other components.
-
Looseness: This refers to the failure of components to remain securely fastened or attached, often caused by wear or vibration.
-
Material Failure: This refers to the failure of materials due to factors such as fatigue, stress, or defects.
-
Mechanical Failure: This refers to the failure of mechanical components or systems, such as bearings or gears.
-
Miscellaneous: This category includes failures that do not fit into any other category, such as those caused by design errors or inadequate maintenance practices.
-
Open Circuit: This refers to failures caused by an interruption in the electrical circuit, such as a broken wire or connection.
-
Out of Adjustment: This refers to the failure of components or systems to maintain the correct settings or adjustments, often caused by wear or improper maintenance.
-
Overheating: This refers to the increase in temperature of components or systems beyond the designed limits, often caused by excessive friction or insufficient cooling.
-
Software Failure: This refers to failures caused by errors or bugs in software used for control or monitoring.
-
Sticking: This refers to the failure of components to move or operate smoothly, often caused by wear, corrosion, or contamination.
-
Vibration: This is a common failure mechanism in turbomachinery caused by the movement of rotating components. Excessive vibration can lead to fatigue failure of components, looseness of bolts and fasteners, and even catastrophic failure of the machine. The causes of vibration can be due to misalignment, imbalance, worn bearings, unbalanced loads, and even electrical or mechanical resonance.
-
Wear: Wear is a gradual process of material loss due to friction or erosion. It is a common failure mechanism in turbomachinery due to the high-speed rotation of components and exposure to high-temperature and high-pressure conditions. Wear can lead to reduced efficiency, decreased output, and increased risk of catastrophic failure. The causes of wear can be due to abrasive particles, corrosion, thermal expansion, or poor lubrication.
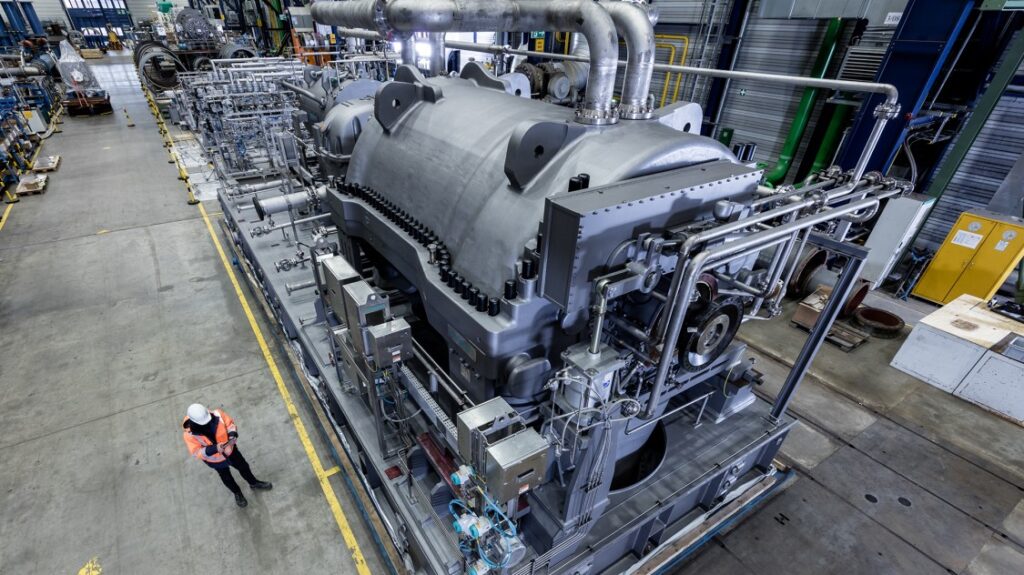
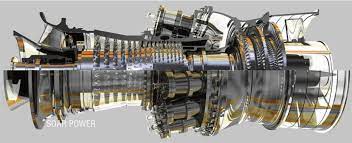
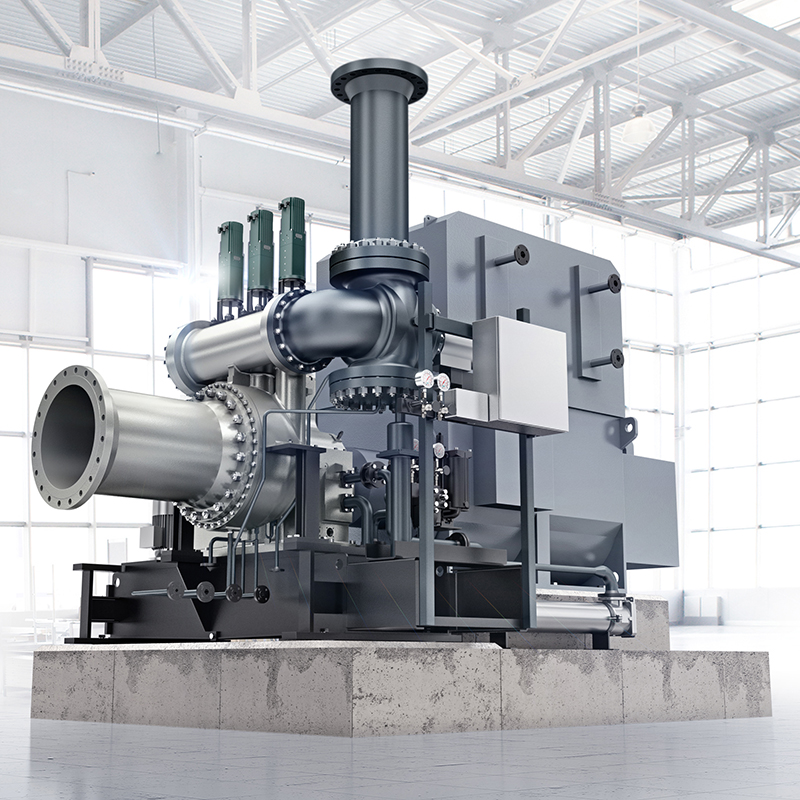